GENETIC TESTING AND ANALYSIS
What is genomic medicine?
​
The National Human Genome Research Institute (NHGRI) defines genomic medicine as "an emerging medical discipline that involves using genomic information about an individual as part of their clinical care (e.g. for diagnostic or therapeutic decision-making) and the health outcomes and policy implications of that clinical use."
​
Genomic medicine evaluates the patient’s illness through a molecular lens to provide patients with a more accurate and effective cure. Genomic medicine has improved the health care of the past. Combining family medical history, diagnosis, and now genetic information, physicians and researchers are able supply the public with precise results, prevention steps, and treatment.
Thanks to the scientists who have endured long hardships in popularizing genomic medicine,we can see genomic medicine improving health all around us:
-
In the United States, every newborn is screened for 29-50 critical genetic diseases.
​
-
Scientists at Stanford University are researching a method to test whether or not a patient’s body will reject a heart transplant using circulating DNA in the patient’s blood to estimate the likelihood of rejection.
​
-
The same circulating DNA is currently being considered as a possible biomarker for specific cancers. After sequencing the circulating DNA, the genetic information can be used to track the development of the tumor.

The first signs of genetic testing appeared in the 1950’s when scientists announced genetic testing was available for cystic fibrosis, Down syndrome, and Duchenne muscular dystrophy. Later, genetic tests gained popularity through required newborn screenings for diseases such as PKU. Today genetic testing serves a variety of purposes from uncovering paternity to guiding prospective parents. The next step in genetic testing is learning to apply it.
In the future, scientists aim to identify all gene’s functions and link them to diseases. Once genetic testing is normalized in healthcare, healthcare providers will be able to pinpoint the cause of the disease with utmost accuracy. Using genomic information as a guide, health care providers will soon be able to use personalized medicine as a form of treatment and prevention. An additional use of genetic testing is gene therapy. Gene therapy is “correcting” genes that cause disease. Currently scientists are developing DNA sequencing technology that will sequence faster than ever before. Modern technology such as DNA chips, mass spectrometry, HPLC analysis, and capillary electrophoresis can lower labor costs which in turn creates more affordable genetic tests. Genetic testing holds such potential, however, the greatest challenge in reaching this future will be trying to protect privacy and prevent discrimination.
Methodologies: How is Genetic Testing Performed?
​
All genetic tests fall into three categories: cytogenetic testing, biochemical testing, molecular testing.
​
Cytogenetic Testing
​
Cytogenetic testing finds and analyzes abnormalities in chromosome structures. Chromosomes consist of compacted DNA and proteins, called histones, found in the nucleus of human cells. Chromosomes, containing the hereditary information, typically have a X structure that easily and accurately separate duplicated DNA used in cell division. Any abnormalities in the chromosomal structure could disrupt the function of the genes located on the chromosome.

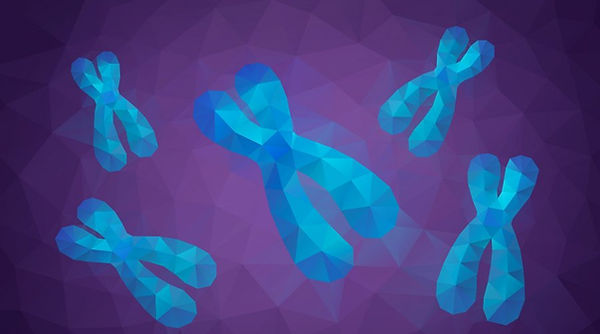
In order to identify abnormalities in chromosomes, samples of blood, bone marrow, saliva, or any other bodily tissues are necessary to perform genetic testing. For blood samples, T- lymphocytes, a type of white blood cell, are extracted and examined for chromosomal defects. Tissues, unlike blood, must be cultured first before staining the chromosomes with dye for easy identification.
Cytogenetic testing can involve a process called FISH or Fluorescent in situ hybridization. FISH uses fluorescent dyes to easily identify any duplications, deletions, translocations, insertions or other abnormalities. FISH uses probes, a piece of DNA or RNA, that attaches itself to a selected sequence or gene. The selected gene is usually a common mutation or problem site. If the patient has the mutation, the probe will attach itself to the site. Since the probe is labeled with a fluorescent molecule, scientists use fluorescent microscopes to quickly determine the mutation’s location.
FISH is used in karyotype and array testing. It is also used to test suspected DiGeorge syndrome also known as velo-cardio-facial syndrome (VCFS) or chronic myelogenous leukemia both commonly found in children.
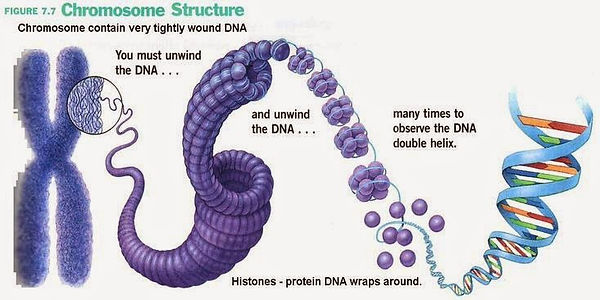
Biochemical Testing
​
Those who suffer from metabolic disorders often choose to perform biochemical testing. Unlike cytogenetic testing and molecular testing, biochemical testing uses proteins instead of DNA. Biochemical testing collects a sample of blood or urine which contains the defective or absent protein. Laboratory workers examine the specific protein’s structure for abnormalities and compare the quantity of protein in the patient to that of normal person using gas, liquid, or tandem mass spectrometry.
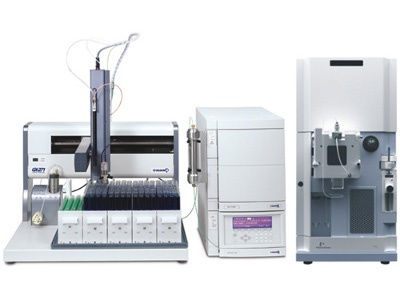
Mass Spectrometer
Molecular Testing
​
Molecular testing is used when the sequence causing the genetic disease is already known. Mostly used for minor DNA mutations, molecular testing involves direct DNA testing. Only a small sample of tissue is required. PCR or polymerase chain reaction, a common biotechnology technique, amplifies the desired DNA sequence. PCR was invented by Kary B. Mullis.
